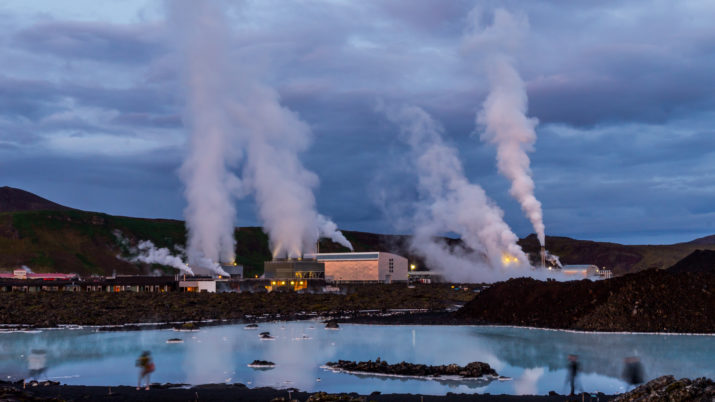
Geothermal Heating and Cooling Networks for Green and Livable Urban Transformations – Part II
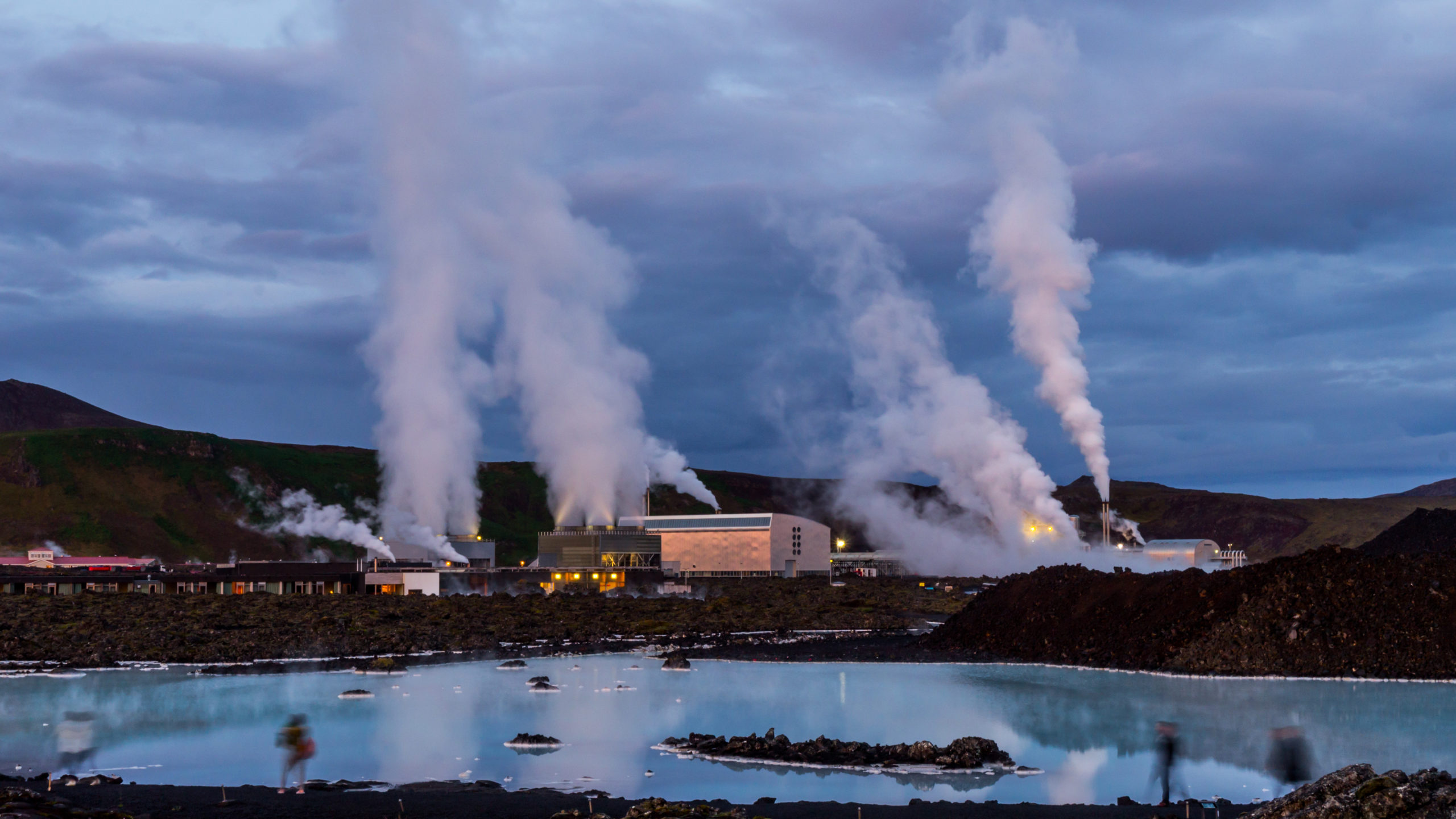
This is part of our special feature, Sustainable European Cities and Digitization.
Click here for Part I.
As stated in part one of “how geothermal heating and cooling networks may support the green and livable urban transformation,” geothermal energy can be very efficiently used as a resource for district heating and cooling networks and can have the ability to be a key technology for a necessary heat energy transition. Compared to natural gas, the reduction of CO2-emissions using geothermal energy in an average project can be up to 88 percent (Stichting Platform Geothermie et al., 2018), hence the implementation of geothermal energy could be an important step to reach EU climate goals. In the Netherlands, for instance, it is predicted that geothermal energy can contribute 15 percent to the necessary CO2-emission reduction in the heat sector by 2030 and up to 25 percent by 2050 (Stichting Platform Geothermie et al., 2018). In contrast to that, geothermal energy only plays a minor role in the European heating and cooling sector. Hence there is a need to strengthen the role of geothermal energy and to intensify a know-how transfer about the potential of this technology. Therefore, the COST Action Geothermal-DHC wants to foster this knowledge exchange and will develop a roadmap towards a better integration of geothermally supplied heating and cooling networks in Europe in the next three years. Showcases and good practice examples offer a reliable option to exchange experiences and achieved success and can incentivize stakeholders to integrate similar solutions in their concepts and strategies. Some of such practice examples, implementing geothermal energy for heating and cooling in various European countries, are described in the following, which show a high variety of application possibilities.
Munich – a European vanguard on large scale geothermal district heating and cooling
The metropolitan area of Munich is an intriguing example of the role that geothermal energy may play in the decarbonization of the heating and cooling sector. Due to its attractive hydrothermal reservoirs, the South-German-Molasse-Basin, it is currently the most active area for deep geothermal energy in Germany. At the moment, more than twenty projects for either sole heating or power purposes or for combined heating and power (CHP) generation can be found (Eyerer et al., 2020 Keim et al., 2020). The installed thermal capacity for district heating purposes in this area is around 300 MWth, which represents around ninety percent of the overall installed capacity of deep geothermal energy in Germany. The brine temperature and the thermal capacity of the existing projects vary in a range of 36 and 152°C as well as 2 and 40 MWth (Keim et al., 2020, BVG, 2019).
The district heating (DH) system of the City of Munich, operated by the City Energy supplier (SWM Services GmbH), is one of the biggest grids in Europe. The local energy suppliers aim at covering the base load of the entire district heating network at a demand level of 400 MWth by renewable sources until 2040, which is a key driver for the deep geothermal energy development (Kröper, 2020). Currently, the Grid is powered by two gas fired CHPs and five geothermal plants. Geothermal sources delivering hot water with temperatures up to 120°C cover generally the base load and the CHPs mainly used for peak load and backup supply. Further geothermal plants will be implemented in the grid to further expand the DH-grid for an increased coverage of the heat demand supply for Munich and to replace the base load part of the gas fired CHPs. Hence, in the future the base load should be covered only by tapping geothermal resources, connecting also geothermal plants in the surroundings of Munich. For this, it is planned to install long transmission systems to transport heat into the city center from existing and future geothermal plants located at attractive geological locations in rural areas located south of Munich (Keim et al. 2020). Currently, around 53 MWth are already installed. A newly built geothermal plant with six drillings and a high productivity of 51 MWth will be implemented in 2021, delivering the heat for around 80,000 citizens. It is combined with an existing gas driven CHP and an absorption pump to deliver also cold to a district cooling grid. Until 2040, an additional fifteen geothermal doublets are planned to be installed. With this setting, the complete base load of the Munich City´s heating grid and at least fifty percent of the total annual heating demand of Munich will be covered in a climate friendly way.
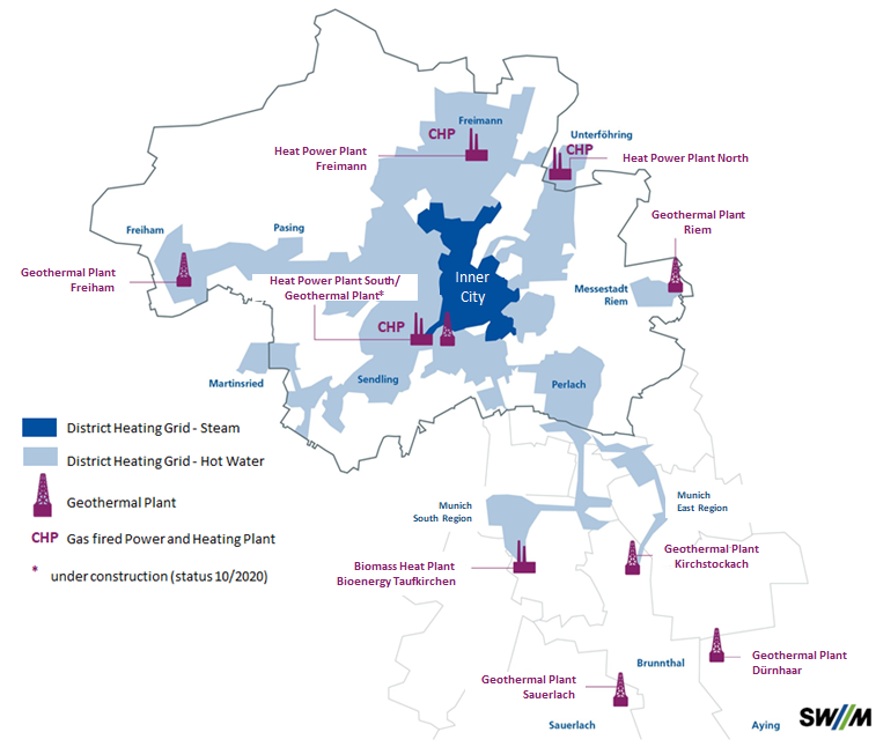
Figure1: District Heating Grid Munich (modified after SWM Services GmbH, Kröper, 2020).
Furthermore, Munich has also an inner-city district cooling grid, operated by the same energy supplier and generally designed in terms of several island networks. These systems will in the final stage include different, locally available cooling sources based on turbo compressors and hybrid cooling units, ice storages as well as adsorption cooling devices based on deep geothermal energy. However, the main source of the cooling grid will be surface water and groundwater, which also represents a shallow geothermal resource. It is implemented to cover the cooling purposes mainly for data centers, office buildings and industrial facilities. At present, six groundwater cooling plants with several wells are in operation producing about 13 MWth. Two additional groundwater plants at a total capacity of around 4 MWth, and an absorption pump plant at a capacity of 35 MWth are under construction. One example of such an island cooling grid is the thermal groundwater use for the research data center of the BMW Group. With 5.34 MWth, the plant covers the cooling base load of the BMW data centers and office building acclimatization. The comparison with conventional cooling techniques shows that direct cooling with groundwater save in this utilization 90 percent of the primary energy, which means about 10 Gwh/a electricity or 6,3000 tons CO2(equ)/a (Arnold, 2006).
Bogatic—the first geothermal district heating network in Serbia
The first geothermal district heating system in Serbia was projected for Bogatic settlement, located in the border area of central Serbia, within the district of Macva. The entire Macva area is recognized as a very potential in terms of geothermal energy: high values of terrestrial heat flow, the existence of the shallow bedded productive geothermal reservoir formed within Triassic limestones and the temperature of the geothermal water at the surface is in the range of 75-80 ℃ (Martinovic, and Milivojevic, 2000).
The usage of geothermal energy in the concept of the district heating system was based on the following premises (Milenic et al., 2016):
- Substitution of the fossil fuels in the existing heating systems in the public buildings
- Increase independence by using the locally available renewables
- Fostering clean and environmentally friendly source of energy
- Sustainable settlement development by decreasing expenses for fossil fuels-based heating systems
- Promoting measures for climate change impact mitigation
The geothermal district heating system has been in operation since the year 2019. The system was projected in the variant of bivalence as an indirect, open geothermal system with cascade use. The installed thermal power of the system is 2.1 MW. The system delivers energy to the seven public utility objects in a total area of 15,000 m2. The five parts of the Bogatic Geothermal-DHC can be considered:
- Exploitation well as a source of geothermal energy
- Distribution pipeline network from well to the users (public utility objects)
- Technical room in objects with heat exchanger
- Technical room with the reservoir for collecting geothermal return water
- Distribution pipeline network for further utilization (second cascade)
The geothermal well is connected with users by a flexible pipeline system in a total length of about 2 km. To ensure minimal temperature losses in the system, the distributive network is built as a pre-insulated pipe made of interpenetrating polyethylene (Rehau, 2008). The operating regime of the system in the first cascade is as follows: primary 75/55°C; secondary 70/50°C (Bogatic Municipality, 2019). At the exit point of the collecting pipeline, water temperature is 50°C with a permanent yield of 25 l/s. Further (cascade) use of the resource is envisaged for various purposes such as heating the central square and supplying the future industrial zone with thermal energy. Used and cooled thermal water disposed into the local surface stream. This option is chosen because of the favorable thermal water chemical composition and balance investment costs of the system.
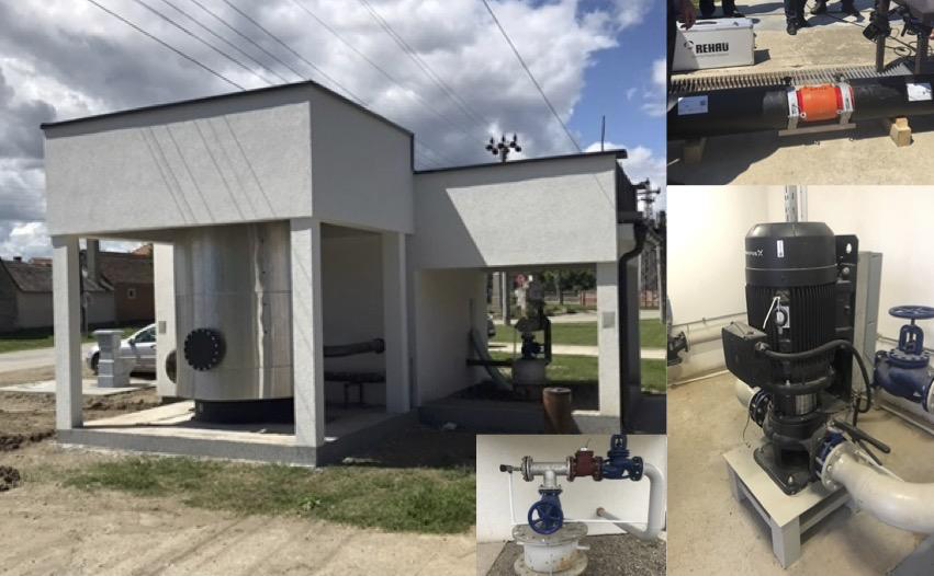
Figure 2: Preview of the Geothermal-DHC system in Bogatic (photo: A. Vranjes, 2019)
Northern Gateway, Colchester, UK – Open Loop District Heating
Northern Gateway open loop district heating system is first of its kind in the UK. It is located at Colchester in county Essex. It is intended to supply heat to homes, offices, and healthcare center at the Northern Gateway development. The district heating system is of 800 kW capacity and will provide 5.5 GWh of low carbon heat per year (Colchester Amphora Energy Ltd, 2021). The system consists of 5 boreholes of 500 mm diameter and 135 m deep (Figure 1) to extract heat from the chalk aquifer lying at 75 m (WJ UK, 2021). The abstraction and injection boreholes are over 550 m distance apart to long term sustainability of the project to extract heat. The ground water extracted from the ground is about 12°C hot which passes through to heat pump system and is then returned to the ground at 5°C (Colchester Amphora Energy Ltd, 2021). Heat will be delivered to houses using underground pipe network and consumption will be measured by heat meters. To ensure commercial viability of the project, heat will be charged by a daily standing charge and per kW of heat consumed.
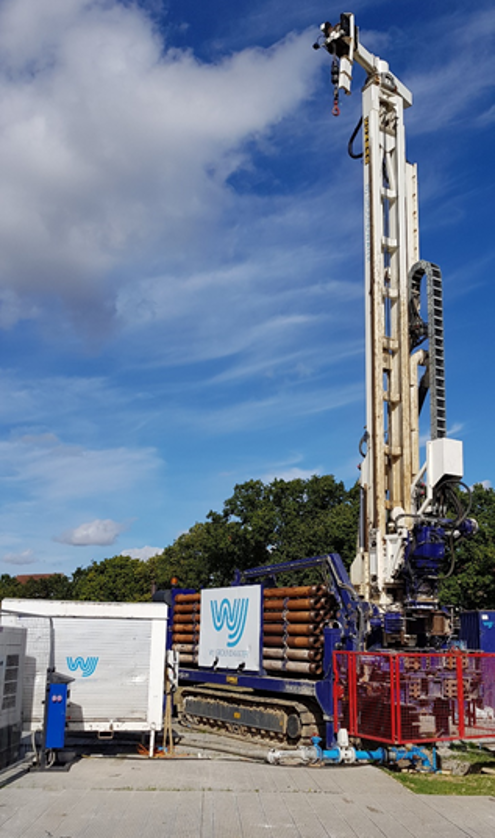
Figure 3. Borehole drilling at Northern Gateway site in Colchester, UK (WJ UK, 2021).
Geothermal heating in northern Italy – the Grado and Ferrara examples
This geothermal pilot project started in 2002 and was completed in early 2015. It is located on the Grado Island in the northern Adriatic Sea (Gorizia Province, NE Italy; figure below), a lagoon part of the Friuli Venezia Giulia Region forming the eastern extension of the Po Valley with unconfined and confined aquifers inside a complex hydrogeological systems with a N-S direction (Della vedova et al., 2015)
This project consists of a geothermal doublet with one production and one re-injection wells. Geophysical surveys were needed in order to drill the Grado 1 Borehole down to 1110 m from the ground, where a terrigenous cover above a Paleogene-Mesozoic basement is present. This was accomplished during the first exploration phase in 2008. During the second phase, in 2014, the Grado 2 borehole was drilled about 1 Km to the East of Grado 1, down to 1200 m from the ground.
Figure 4: The Grado Locality (left side, top) in the North-Eastern part of Italy as highlighted by the dark blue circle in the map (on the right side) and the Ferrara Locality (left side, bottom) in the Central-Northern part of Italy as highlighted by the red circle in the map (on the right side).
Pumping tests and numerical thermo-fluid dynamic simulation in order to verify the reservoir characteristics and the hydraulic connection between wells, as detailed reported in Della Vedova et al., (2015). This allowed understanding also how to project the final design, and to optimize and manage the district heating geothermal plant. Horizontal directional drillings under the port canal downtown were hence designed and realized for the heating distribution network. The planned drilling length was about 2Km long but later further extended. The geothermally supplied district heating network was designed to operate six months per year, twelve hours per day, ensuring cost savings of about EUR 80,000 to 100,000 per year at a capacity factor of 0.2, which could be more sustainable increasing up to 0.4 or even more.
The second example on geothermal heating use is located in the Ferrara Municipality (Central Northern Italy, figure below) inside the Emilia-Romagna Region, which stretches from the Apennine Ridge to the Po River and the Adriatic Sea. This plant consists of a geothermal District Heating and Cooling system which supplies about 320 buildings for the heat producing and about eighty-five buildings for sanitary hot water consumption. This plant is integrated with a biomass plant for solid wastes and methane gas boilers as back-up. The geothermal plant consists of two geothermal production wells and one reinjection well reaching depths of approximately 1,000 m below surface to trap a geothermal aquifer at temperatures of around 100°C. The overall thermal demand of 170 GWh is covered by a combined 29 MWt CHP (Combined Heat and Power plant), supplied by municipal solid waste and by auxiliary natural gas boilers. A short-term thermal energy storage at a capacity of 45MWh decouples the heat production plants from the distribution network. It was further implemented by an advanced control monitoring system in order to give a high-level service to the customers connected to the distribution energy network and searching for the maximum electricity saving (Manente et al., 2019).
As detailed reported in Manente et al., (2019), experimental data measured in the first months of plant operation, show a high saving potential of electric power for pumping equal to 400 MWh per year, which represents a saving of 30 percent which can be improved thanks to the enhanced storage operation. This further feature enables a saving of 7000 MWh per year of energy from natural gas. The calculated payback time over few years shows the high-cost saving potential in such systems.
Using case studies to close knowledge gaps about geothermal energy
All these good practice examples showcase the wide range of use of geothermal energy with different geothermal technologies, under different conditions and in different scales but with the same aim to cover the heating and cooling demand in a climate friendly way and to reduce CO2-emmisions. By collecting, analyzing and categorization of projects as shown above and supported by further stakeholder interactions as questionnaires and interviews, the COST Action Geothermal-DHC wants to derive a user-tailored FAQ catalogue for stakeholders related to the implementation of geothermal energy into district heating and cooling networks. This information should close knowledge gaps and overcome concerns about geothermal energy technologies and raise the attention for the possible high contribution of geothermal energy to achieve the climate goals. For more information on Geothermal-DHC please visit www.geothermal-dhc.eu.
Kai Zosseder, Dr., is Head of the Geothermal Group of the Chair of Hydrogeology at the Technical University of Munich. He has been working since 2010 in the field of Geothermal Energy, leads many projects concerning Geothermal Energy Developments and is Executive Member of the EU COST Action CA18219 Geothermal-DHC.
Ana Vranjes, Dr., is an Assistant professor in the Department of Hydrogeology at the Faculty of Mining and Geology, University of Belgrade. She is a leading researcher in the field of geothermal energy at the UoB, actively involved in numerous national and international geothermal projects, including EU COST Action CA18219 Geothermal-DHC.
Jessica Chicco, Dr., is a Post Doc at the University of Turin (Italy). She is working on different projects concerning low enthalpy geothermal energy for direct uses as well as underground thermal energy storage systems. She is currently involved in the EU COST Action CA18219 Geothermal-DHC as co-leader of PWG1 about Technology, and Leader of the AdHocWG2 about underground storage systems.
Rao Martand Singh, Dr., is a Professor in the Department of Civil & Environmental Engineering at NTNU (Norwegian University of Science & Technology). He has been working in the area of energy geotechnics for last 12 years, mainly focusing on geothermal energy structures such as energy piles and energy tunnels. He is Executive Member of the EU COST Action CA18219 Geothermal-DHC.
Gregor Goetzl, MSc, is a senior researcher on geothermal energy use at the Geological Survey of Austria. Since 2019, he is chairing the EU COST Action CA18219 Geothermal-DHC, which focuses on the integration of geothermal energy into heating and cooling networks.
The case studies described here are used for knowledge exchange in the currently operating COST Actions CA18219 Geothermal-DHC. COST is a European research funding program under the Horizon 2020 framework programme to support the promotion of scientific excellence.
Click here for Part I.
References
Arnold, M. (2006). „Einsatz von Fernkälte aus Grundwasserüberleitungen für ein Rechenzentrum in München“. Accessed April 19, 2021. https://www.swm.de/dam/doc/geschaeftskunden/fernkaelte/abschlussbericht-fernkaelte-dt.pdf
Bogatic Municipality. 2019. “Technical documentation on the geothermal district heating system of the public utility objects in Bogatic”. Fund documentation.
Bundesverband Geothermie e.V. (BVG) (2019). „Geothermie in Zahlen (2019)”. Accessed October 27, 2020. https://www.geothermie.de/geothermie/geothermie-in-zahlen.html.
Colchester Amphora Energy Ltd. 2021. “Northern Gateway Heat Network”. Accessed April 19, 2021. https://amphora-energy.co.uk/
Della Vedova B., Cimolino. A., Castelli E., and Brancatelli G. (2015). Geothermal Heating and Cooling in the FVG Region: the Grado District Heating and the Pontebba Ice Rink Plants. Proceedings and AdrJo.Platform, Losinj 2014 Workshop on Geothermal Energy, pp. 65-76.
Eyerer, S., Schifflechner, C., Hofbauer, S., Bauer, W., Wieland, C., and Spliethoff, H. 2020. „Combined heat and power from hydrothermal geothermal resources in Germany: An assessment of the potential”. Renewable and Sustainable Energy Reviews.
Keim, M., Loewer, M., Molar-Cruz, A., Schifflechner, C., Ferrand, T., Wieland, W., Kuhn, P., Bohnsack, D., Heine, F., Konrad, F., Pfrang, D., Schölderle, F., Zosseder, K., Bauer W., Drews, M., and Hamacher, T. 2020. „Bewertung Masterplan Geothermie Bayern“. Geothermal-Alliance-Bavaria.
Kröper T. [2020). „Integriertes Wärmenutzungskonzept am HKW Süd in München Sendling“. Praxisforum Geothermie.Bayern 2020.
Manente G., Lazzaretto A., Molinari I.,and Bronzini F. (2019). Optimization of the hydraulic performance and integration of a heat storage in the geothermal and waste-to-energy district heating system of Ferrara, Journal of Cleaner Production, Volume 230, pp 869-887, ISSN 0959-6526, https://doi.org/10.1016/j.jclepro.2019.05.146.
Martinovic, M., and Milivojevic, M. 2000. “Hydrogeothermal model of Macva”. Proceedings World Geothermal Congress 2000, Kyushu – Tohoku, Japan, May 28 – June 10.
Milenic, D., Vranjes, А., and Stanic, N. 2016. “The Study of possible hydrogeothermal energy use for the sake of district heating of public utility objects in Bogatic”. The Faculty of Mining and Geology, Belgrade
Rehau. 2008. “Insulpex – a flexible piping system for a district supply with thermal energy: Technical information 78.600/2 RS”.
Stichting Platform Geothermie, DAGO, Stichting Warmetenetwerk, and EBN. 2018. “Master Plan Geothermal Energy in the Netherlands – A broad foundation for sustainable heat supply.
WJ UK. 2021. “Colchester Northern Gateway project”. Accessed April 19, 2021. https://wjgl.com/featured-projects/colchester-northern-gateway/.
Photo: Svartsengi Geothermal Power Plant in Iceland steaming massively with reflection in a blue water of geothermal pool after the sunset and dramatic blue violet sky with thick clouds | Shutterstock
Published by May 11, 2021.