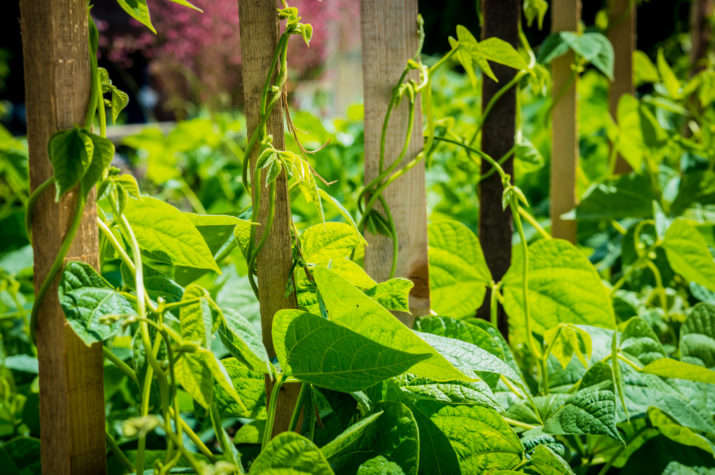
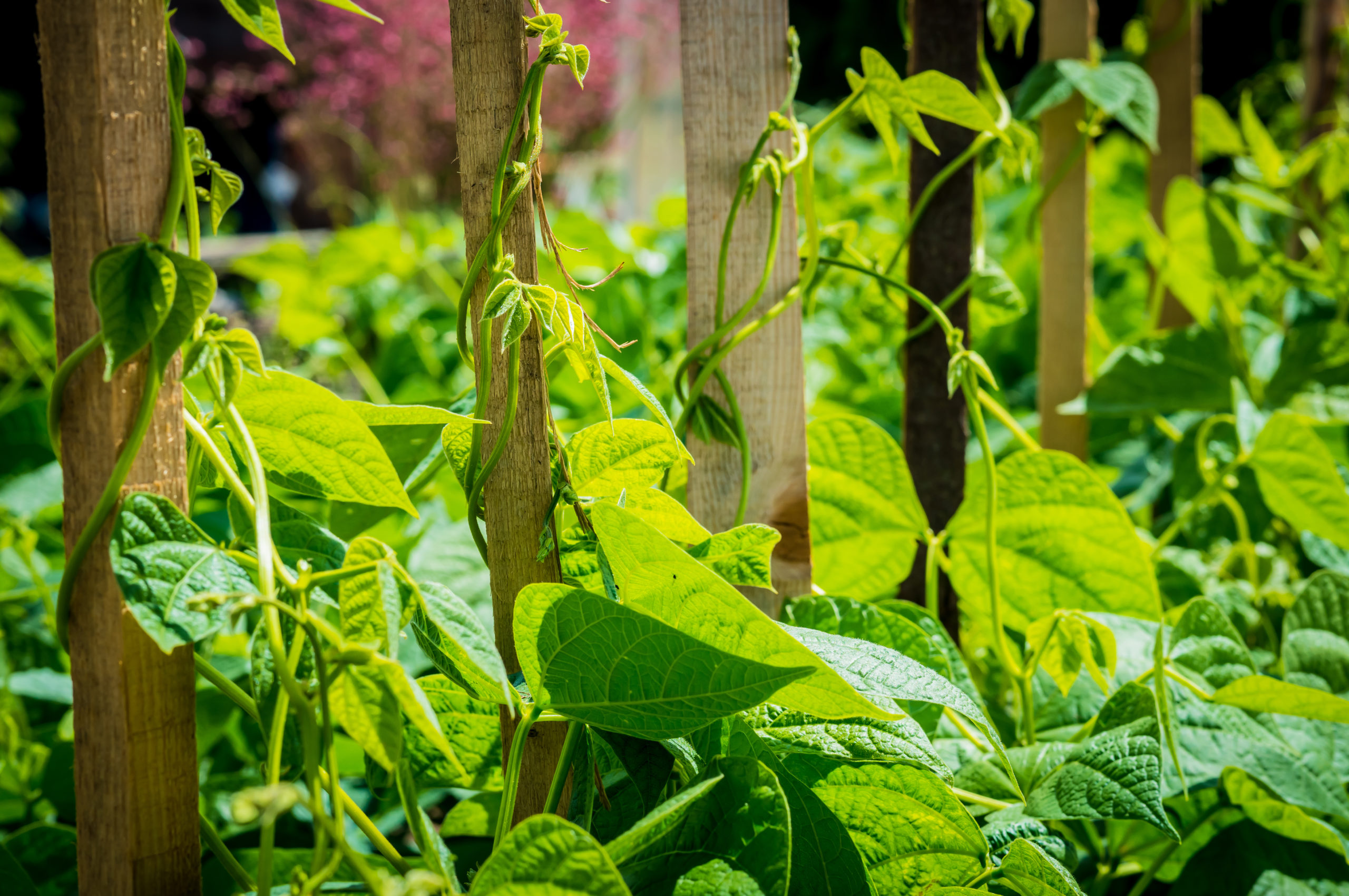
This is part of our special feature, Rethinking the Human in a Multispecies World.
The idea that most plants move on their own is somewhat uncommon. We all have seen a Venus fly trap catching an insect or maybe a Mimosa pudica closing its leaves after being touched. However, for the most part, we tend to see plants as the static background of our lives. Plant blindness refers to a general attitude that regards plants as if they were merely part of the landscape [1]. Plant blindness advances that humans simply do not see plants as they see animals. For example, in some places, we do not study them in high school biology classes as much as we do animals. In a way, this is to be expected since both evolutionarily and behaviorally, humans are closer to animals than to plants. Indeed, even when an animal is very different from us, it is still closer to us than almost any plant. One factor contributing to this contrast is the way we relate to movement, which is central to the way humans experience their surroundings. As humans, we move to perceive, and in turn, movement is one of the main features of the perceived environment [2]. Like us, animals locomote and change their posture. And they do so at a pace that our perceptual systems easily notice. However, we cannot perceive movement at all temporal scales. If the movement is too fast or too slow, our perceptual systems are unable to detect it. An event appears to be instantaneous if it moves too fast, or still if it moves too slowly. Most plants fall in the latter category: even though they move pervasively, their movement is too slow for us to notice it. For this reason, we do not usually regard plants as moving.
The belief that plants do not move is plainly mistaken. We know some plants like the Venus fly trap and the Mimosa pudica move and actually do so rather fast. In the case of the Venus, for instance, it moves fast enough to trap an insect within its leaves. At least since Charles Darwin’s works [3], we know that many other plants move even if at a slower pace. In presenting some key properties of plants’ movements, I focus on an oscillatory movement called nutation (or circumnutation) that can be observed in many vines, such as the common bean (Phaseolus vulgaris) or pea plants [4]. I choose nutation because it is a quite common movement that many species exhibit. Nutation makes the movement of plants typical rather than exceptional.
Going round, and round, and round
In some ways, the slow movements of plants are similar to the movements of stars. If one looks at the stars in Spain or anywhere in the Northern hemisphere at night from a place with little pollution, it is possible to see a seemingly static pattern. However, if one were able to look at that pattern for long enough and with enough attention, it would not take too long to observe that stars are constantly moving in the sky. Indeed, this has been a well-studied phenomenon since ancient times. In our example, looking at the stars from Spain reveals their pattern of rotational movement around the Pole star. This rotational movement entails a pattern displacement of about fifteen degrees per hour, which is equivalent to a quarter of degree per minute. However, as clear and well-known as this movement may be, most of us cannot see it with the naked eye. We need technological assistance, such as time-lapse videos, in order to notice the stars’ rotation. We are unable to notice such an ostensible, open movement because humans’ visual system cannot perceive change at such slow temporal scale, akin to its inability to perceive minute elements along parts of the light spectrum. There are several reasons why our visual system is unable to perceive slow changes, spanning from physiological limitations to evolutionary pressures. As an example, most aspects of the environment we need to perceive for a successful engagement with our surroundings are not super-fast or super-slow, but happen at a middle speed: animals walking, faces moving while speaking, water being poured in a glass, etc. All these are neither fast nor slow and constitute illustrations of the usual events we face in our environments. Our perceptual systems are therefore tuned to perceive events at these velocities, but unable to do so for events that occur at much faster or slower speeds [2].
Along with being very slow for human perception standards, like the movement of stars, plant nutation is rotational. The movement of nutation consist of the bending of the shoot tip of the plant that, when observed over time, describes a rotational, oscillatory movement (see Figure 1) [4]. Each one of the revolutions of the oscillatory patterns of nutation may last between two or three hours for plants like climbing beans. In this sense, nutation is not as slow as the rotation of the stars, but it is still too slow to be noticed by visual systems like ours.
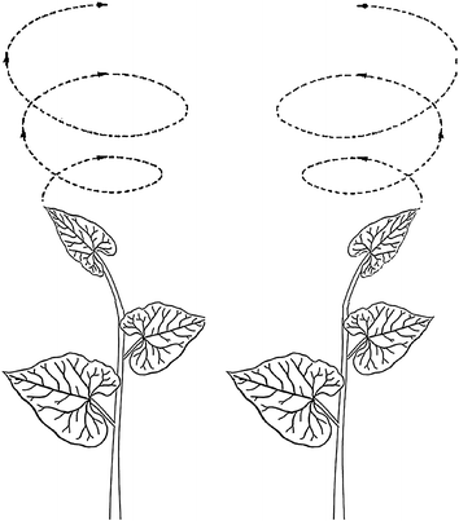
Figure 1. Schematic example of nutation. We can see two different shoots and the arrows illustrate the movement of nutation for each: one clockwise (left) and the other counterclockwise (right). Picture appears in [4].
Although nutation has been described since at least the late nineteenth century [3], recent technologies, like time-lapse photography and video, have allowed us to observe it in ways that accommodate our own capacities better. In addition to this new form of observation, such recent technologies help us gather new data about nutation in order to better understand the phenomenon.
In the context of the new understanding of nutation scaffolded by those new technological means, an old question re-emerges: is nutation a pure hardwired/reactive movement or is it a controlled and flexible movement instead? In other words, we can take nutation to be as the sun-tracking behavior of the sunflower, that is, a purely reactive behavior to one environmental cue (the position of the sun) that the plant is not able to control. Or we can take nutation to be a controlled behavior, more akin to the behavior of animals, that plants can flexibly control in order to cope with their environments. The latter was the position Charles Darwin defended and that some plant scientists still endorse [5]. The main idea is that plants can use nutation as a means to accomplish goals in their environment, such us finding a support to climb―in the case of climbing beans.
Plant nutation, metalheads, and metal balls
To explore the idea of whether nutation is a controlled, flexible behavior that plants use to accomplish goals in their environments, we designed a study in which we recorded the movement of several pairs of climbing bean plants in identical environments [6]. The only difference between the plants in each pair is that one of them had a support to climb (a pole) in its vicinity. The recording was performed with time-lapse cameras and identical environments were achieved by constructing two recording booths for which we were able to control light, temperature, humidity, etc. (Figure 2).
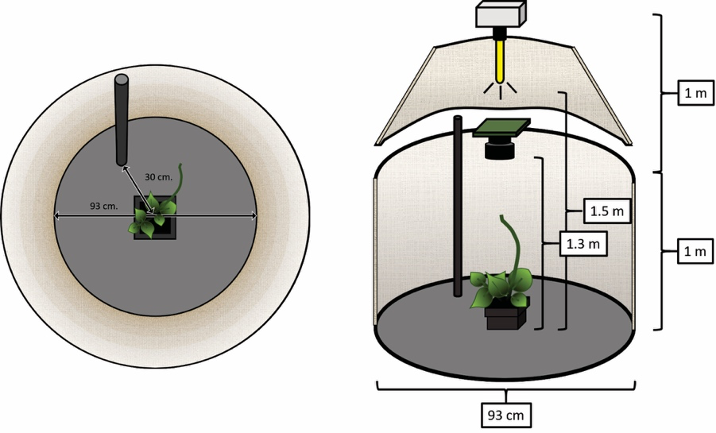
Figure 2. Schema of the recording booths for the experiment in [6]. The figure shows the zenithal (left) and lateral (right) views of the booths in which the plants had a support to climb (pole) in their vicinity. The booth without the support to climb is identical to this one.
Our hypothesis for this experiment was quite simple: assuming that finding a support to climb is a fundamental goal of climbing beans in their environments, if the plants control their nutation patterns to achieve such a goal, having a support to climb in their vicinity must have an effect on those patterns. To test this effect, we analyzed the complexity signaturesof the movement of the climbing beans. We know these signatures can help us to distinguish between qualitatively different kinds of movements. Concretely, the difference between the simple motion of physical systems and the more complex motion of the controlled, goal-directed behaviors of biological systems. But how can complexity signatures of movements help us in doing so? A good illustration may be the difference between falling metal balls and walking metalheads.
In a famous myth of the Scientific Revolution, Galileo Galilei climbed the tower of Pisa in order to disprove Aristotle’s theory of falling bodies. Put simply, Aristotle thought the weight of objects influenced their falling speed. On the contrary, Galileo hypothesized that bodies of similar shape and size would fall equally fast despite having different weights. To test this hypothesis, Galileo let two balls of similar shape and size, but different weight, fall from the tower: a wooden ball and a metal ball. The well-known myth ends with the two balls falling at the same speed, therefore corroborating Galileo’s hypothesis. Beyond this result, the most interesting aspect of this myth for our aims is to imagine the falling of, say, the metal ball. We can imagine Galileo dropping the metal ball from the tower. We all would agree that the ball would fall in a straight line from its initial position to the ground of Pisa—granted there are no other forces (like strong wind) influencing the movement. Moreover, if the metal ball were thrown again and again by Galileo from the same position, it would fall in exactly the same way every time. In this sense, the falling of the metal ball from the tower of Pisa tower would exhibit a highly predictable movement with very low variability—literally, a straight line. The combination of these two features is a typical signature of non-complex movements. Typical movements of physical bodies usually exhibit this signature.
Compare the falling of the metal ball in the Galilean myth with a metalhead walking through the city to attend a heavy metal concert. The metalhead will depart from some place in the city and will arrive at the concert venue. On the way there, the metalhead will take hundreds or thousands of steps, change direction, cross streets, and so on. What is the difference between the movement of the metalhead and that of the metal ball? The simplest answer to this question is that, while the metalhead had a goal and engaged in a behavioral organization (walking) as to accomplish that goal, the metal ball had no goal and just fell to the ground following the basic laws of physical motion. But how is this difference manifested in movement itself? To answer this question and replicate Galileo’s strategy of dropping the metal ball from the tower of Pisa repetitively, we can imagine that the metalhead keeps coming to the concert venue again and again to attend different events. Even when following the exact same route, same streets, same crosswalks, and so on, every time the metalhead walks to the venue will be slightly different from the previous: the crossing of a street will not happen at the same precise spot every time, each step taken will be slightly different from the others, the velocity of the walking will be different at any instant, etc. However, the ways to the venue will still be similar to each other. In this sense, the behavior of the metalhead would exhibit a fairly predictable walking pattern with very high variability. In a way, the walking of the metalhead constantly changes in the smaller scales (steps, turns, etc.) to remain rather the same during the overall route. The combination of these two features, variability and stability at different scales, is a typical signature of complex movements. Typical movements of biological and cognitive systems usually exhibit this signature.
The differences between the movement of metal balls and metalheads stand as succinct illustrations of the way in which complexity signatures helped us to test our hypothesis regarding the movement of nutation in climbing beans plants. We thought that, if our hypothesis was right, the movements of the bean plants should exhibit the complexity signatures of goal-directed movements—similar to the movements of the metalhead walking. And that was exactly what we found in the results of the study [6]. The movements of nutation of the bean plants that had a support to climb (a pole) in their vicinity were significantly more complex than those of the bean plants that did not have the support to climb near them. In other words, in terms of its complexity signatures, the movements of nutation in climbing bean plants seem to be constituting a goal-directed behavior and, as Darwin proposed, could be an example of controlled, flexible behavior that plants can exhibit in order to cope with their environments.
Plants movement in a human world
Our study shows that the movements of plants may be more intricated and nuanced than it is usually acknowledged. The patterns of nutation of climbing beans when looking for a support to climb in their vicinity clearly show that goal-directedness is a plausible phenomenon in the plant world. That is, climbing beans may be actively exploring their environment to find the best conditions for their own development: the best growing environment, the best light, etc. And the complexity signatures of their movements are a way in which they show such goal-directedness. It is a compelling scientific result because to show that (at least some) plants are able to exhibit goal-directed behaviors to cope with their environments may have dramatic implications for our understanding of plants as biological systems, but also as cognitive [7], or even sentient [8] systems. However, these implications are not straightforward and cannot be supported by a mere handful of studies pointing to their plausibility. In a human world, plant movement requires further research if it is going to be pivotal in understanding the most complex questions of plant life.
Moreover, plant life goes beyond basic scientific research. Aside from questions about movement, there are other urgent questions to be asked regarding sustainability, agricultural techniques, and our general relationship with plants. In facing contemporary climate challenges, our societies are looking for more sustainable and effective ways to engage with the planet. One of the most stimulating ways of doing so is to find more sustainable, more effective ways to grow plants, which would lead to less space devoted to agriculture, less resources consumed, and more resources for less developed regions. A new approach to plant movement provides a better understanding of how plants cope with their environments through movement and allow us to better design agricultural environments that are fit for their development. This would be a natural way to potentiate their growing and, generally, to improve the sustainability and efficiency of our agriculture. This kind of approach is already being explored in terms of other features of plant physiology—for instance, the use of Phytomelatonin in reducing the environmental stresses of plants [9]—but the focus on plant behavior, and plant movement in particular, is still underdeveloped. Acknowledging the possibility that plants have a rich behavioral repertoire, such as movement, is one way to address the sustainability question in agricultural environments. In this sense, plant movement is an open window to a better relationship between plant life and our human world.
Vicente Raja is a postdoctoral fellow at the Rotman Institute of Philosophy (Western University, Canada). He studies philosophy, cognitive science, and theoretical neuroscience paying special attention to embodied approaches to brain activity and behavior. His experimental work encompasses nonlinear analyses of human and plant behavior.
References
[1] Jose, SB, Wu, C-H, Kamoun, S. 2019. Overcoming plant blindness in science, education, and society. Plants, People, Planet, 169– 172. https://doi.org/10.1002/ppp3.51
[2] Gibson, J. J. The ecological approach to visual perception (Houghton Miffin, Boston, 1979).
[3] Darwin, C. A. The Movements and Habits of Climbing Plants (Murray, Sydney, 1875). Darwin, C. A. & Darwin, F. The Power of Movement in Plants (Murray, Sydney, 1880).
[4] Mugnai, S., Azzarello, E., Masi, E., Pandolfi, C. and Mancuso, S. Nutation in plants. In Rhythms in Plants (eds Mancuso, S. & Shabala, S.) 19–34 (Springer, Berlin, 2015).
[5] Stolarz, M. 2009. Circumnutation as a visible plant action and reaction. Plant Signaling and Behavior 4: 380–387.
[6] Raja, V., Silva, P.L., Holghoomi, R. et al. 2020. The dynamics of plant nutation. Scientific Reports 10, 19465. https://doi.org/10.1038/s41598-020-76588-z
[7] Trewavas, A. 2017. The foundations of plant intelligence. Interface Focus 7, 20160098. https://doi.org/10.1098/rsfs.2016.0098
[8] Raja, V., and Segundo-Ortín, M. 2021. Plant Sentience: Theoretical and Empirical Issues, Editorial Introduction. Journal of Consciousness Studies 28(1-2), 7-16.
[9] Altaf, MA, Shahid, R, Ren, M-X, et al. 2021. Phytomelatonin: An overview of the importance and mediating functions of melatonin against environmental stresses. Physiologia Plantarum 172, 820– 846. https://doi.org/10.1111/ppl.13262
Photo: Bean sprouts in the garden | Shutterstock
Published on November 9, 2021