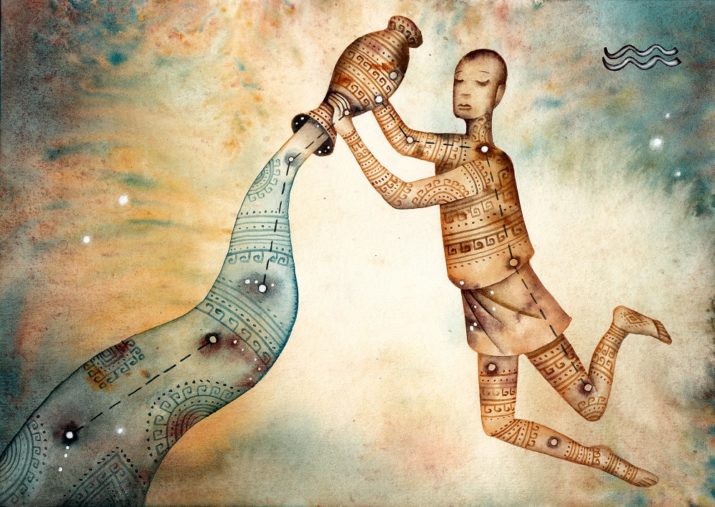
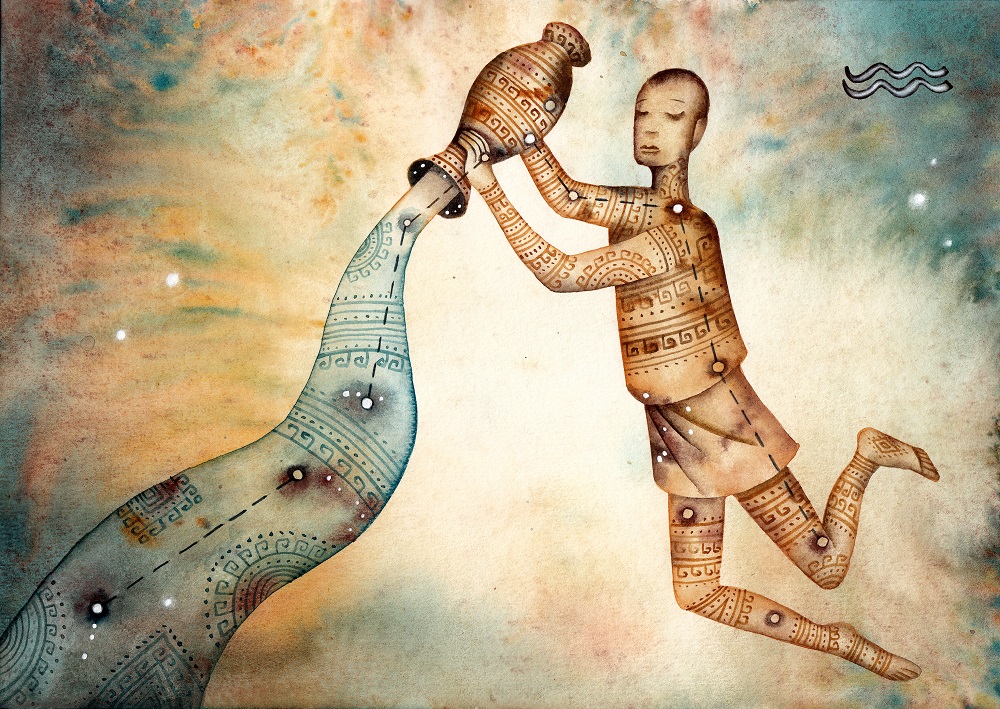
This is part of our special feature on Water in Europe and the World.
Water has always been essential for human societies providing ecosystem services, such as drinking water, crop production through irrigation water, food, climate regulation, and recreation. The German sociologist Karl Wittfogel went so far as to suggest a connection between water and the evolution of the state, the so-called “hydraulic societies.” Today, water management is a key sector of environmental policy in Europe, and arguably the environmental sector with the highest aspirations. The Water Framework Directive (WFD), Europe’s main legislation in the field of water policy that was adopted in 2000, aims at reaching at least a good chemical, quantitative, and ecological status in European water bodies until the end of the next decade (EC 2000).
The Directive has resulted in extensive monitoring programs by the European member states, covering groundwater and surface waters. Several large European research projects have focused on the development and standardization of tools for a European water management (e.g. Hering 2006, Schmidt-Kloiber 2012). Depending on the water body type and monitoring program, between 28 percent and 86 percent of large European Water bodies have been monitored with a total of over 130,000 monitoring sites, providing a rich data set to assess the status of Europeans water bodies (EEA 2018). Groundwater systems are assessed for their quantitative and chemical status, surface waters for their ecological and chemical status.
The quantitative status is regarded as good if the level of water abstraction does not reduce the groundwater resource. The chemical status is regarded as good if the concentrations of selected toxic chemicals do not exceed thresholds considered safe for the environment and human health. Finally, the ecological status is regarded as good or better if metrics related to biota—such as algae, invertebrates, and fish—meet specified values. However, the ecological status is also determined by the quality of the habitat for the freshwater organisms in terms of hydromorphological and physicochemical variables, including toxic chemicals, nutrients, barriers, and pH. Irrespective of the values for biological metrics, a water body fails the good ecological status if the values of the hydromorphological or physicochemical variables miss certain targets. Interestingly, toxic chemicals contribute to both the ecological and chemical status assessments. The rationale is that the chemical status is based on a set of compounds, so called priority pollutants, that all member states are required to measure, i.e. which are considered as relevant European-wide. By contrast, the toxic chemicals considered for the ecological status are selected specifically for individual river basins.
Missing the goals
Overall, the status of European water bodies is a long way from meeting the aspirations of the WFD, though this varies with water body type. Groundwater bodies are in a reasonably good shape with 89 percent achieving a good quantitative status and approximately 3 of 4 have a good chemical status, i.e. the concentrations of toxic chemicals are below the thresholds (EEA 2018). The main culprits for failing the good chemical status of groundwater are nitrate and pesticides, mainly from agriculture. The situation in surface water bodies is much grimmer. Only approximately 40 percent each meet the good chemical and ecological status (Figure 1).
The failure for surface water to reach the good chemical status is predominantly due to a handful of toxic chemicals, such as mercury, cadmium, polybrominated diphenylethers (PDBEs), and polycyclic aromatic hydrocarbons that are more or less ubiquitous. Except for PDBEs, they originate mainly from combustion processes, e.g. burning of fossil fuels and waste. PDBE pollution largely represents a legacy of their past use as flame retardants, the dominant PDBEs have been banned internationally (Fantke et al. 2015). If omitting these chemicals from the assessment, only 3 percent of water bodies would fail to meet a good chemical status.
The reasons for the failure to meet the good ecological status are much more diverse and complex. Based on the assessment by the European Environment Agency (EEA 2018), mainly hydromorphological degradation and nutrients are responsible for not achieving a good ecological status. Toxic chemicals that occur in individual catchments were only responsible for 5 percent of water bodies failing to achieve a good ecological status. However, this figure may be misleading given that a study on the pollution by toxic organic chemicals of European streams and rivers identified almost half of the streams and rivers at ecological risk from chemicals (Malaj et al. 2014). This discrepancy between both assessments can be explained by two reasons:
First of all, of the 44 chemicals that drove the risk in the study of Malaj et al. (2014) only 12 were assessed with respect to the ecological status and only in some European member states. Moreover, the study of Malaj et al. (2014) relied on ecotoxicological risk thresholds, derived largely from scientific studies, whereas the ecological status assessment relies on regulatory thresholds that do not necessarily match with these.
A detailed study for Germany also highlighted that other stressors than hydromorphological degradation and nutrients may pose a bigger problem than currently perceived (Schäfer et al. 2016): Hydromorphological degradation and nutrients exceeded risk thresholds at approximately 85 percent of the sites in rivers, but the two other stressors assessed, toxic organic chemicals and invasive species, also occurred above risk thresholds in every second sampling site. Indeed, the failure to achieve at least a good ecological status is clearly a multiple stressor issue. Schäfer et al. (2016) reported that in more than 80 percent of the sites more than two of the four stressors assessed occurred above risk thresholds. Similarly, assessing the effects of multiple stressors on fish in European rivers, another study found that 88 percent of impaired sites were affected by two or more stressors (Schinegger et al. 2016). Hence, an improvement of the ecological status clearly requires consideration of multiple stressors.
Lack of harmonization prohibits comparisons among European states
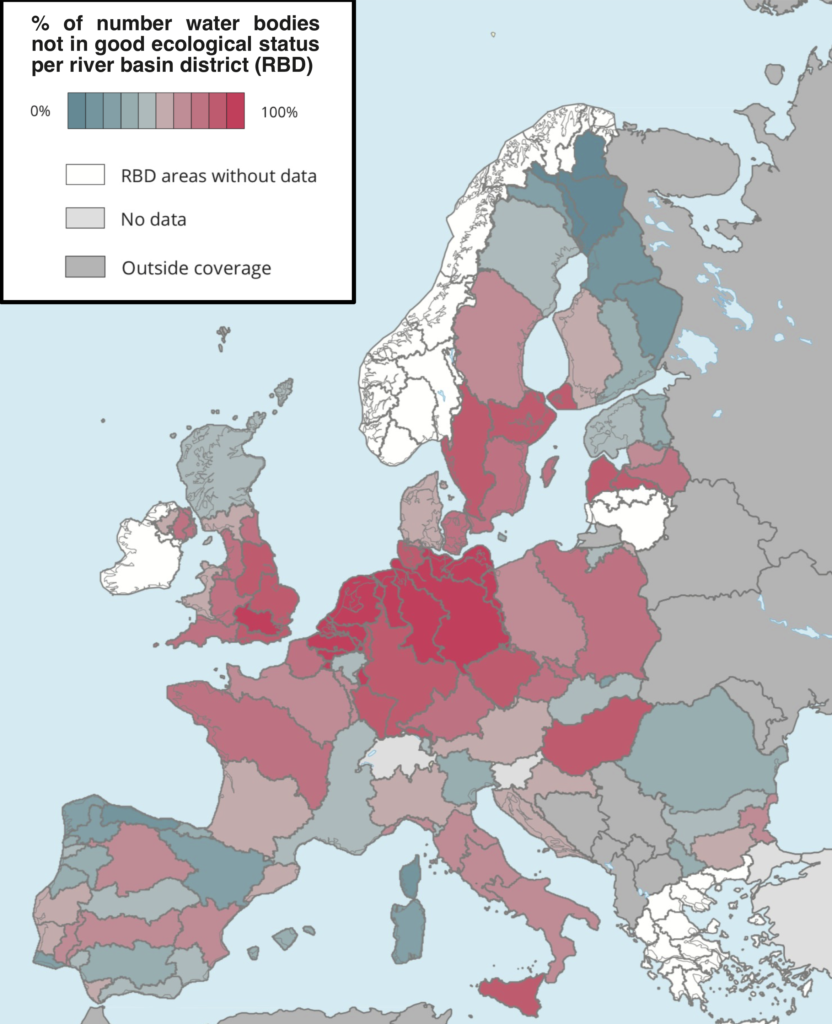
Figure 1: Ecological status of European water bodies. Modified from EEA (2018).
Looking at figure 1 suggests that the failure to meet the ecological status is particularly a challenge in central Europe. However, such comparisons are misleading because of many differences in the methods and monitoring strategies.
For example, the resolution in terms of the number of sampling sites and frequency of monitoring varies strongly between states (e.g. see Malaj et al. 2014).
Also, the assessment of the ecological status based on biological metrics varies with the metric. For example, the newly developed I2M2 metric for France is much more efficient in the detection of impaired reaches than an older metric (Mondy et al. 2012). Unless similarly efficient metrics are used in the assessment for all member states, a better status may be due to a less efficient metric.
The example of toxic chemicals also illustrates the lack of harmonization. The number of toxic chemicals considered as a pollutant for a specific river basin varies between 3 and 151 for rivers, and the variability is equally high for transitional waters, coastal waters, and lakes (Vorkamp & Sanderson 2016). This means that the status can simply be better, because less compounds have been considered, which has been confirmed in the study of Malaj et al. (2014). Even if two member states consider the same toxic chemical as a pollutant for a specific river basin, the concentrations that should not be exceeded can differ by several orders of magnitude between states. For example, in extreme cases like the herbicide bentazone, the threshold concentrations differ by a factor of up to 5000 between member states (Vorkamp & Sanderson 2016). Hence, also the European Environment Agency warns that the status is not that easily comparable between member states unless a further methodical harmonisation has been achieved (EEA 2018).
Water Framework Directive and the Clean Water Act
The US Clean Water Act (1972; CWA) has similarly high aspirations as the European WFD, for example, to restore and maintain the physical, chemical and biological integrity of all water bodies. These aspirations include the goals to discharge of toxicants below levels at which they are toxic, to achieve zero discharge of (point-source) pollutants and to attain a water quality that makes water bodies swimmable and fishable by 1985 and 1983, respectively (Adler 2017). However, these goals have not been achieved until 2018 (Adler 2017). Hence, the CWA and the WFD are similar with respect to the aspirations and the lack of considerable progress in the last two decades. Notwithstanding, the WFD has clearly a much more refined methodology to foster progress and has set clear deadlines as to when to achieve the good status. Currently, the WFD is reviewed and it will be seen whether these deadlines will be adjusted or enforced more strictly.
How to improve the status?
The main focus of improving the status of surface water bodies has been on improving hydromorphological quality, e.g. through restoration measures such as the removal of river bed sheeting, dams and weirs, the introduction of dead wood or other structures increasing habitat complexity, re-allowing the meandering of rivers, and reducing the input of nutrients (Palmer et al., 2010; Borchardt et al. 2013). However, neither the chemical nor ecological status has seen much improvement over the last decade (EEA 2018). Multiple reasons can explain this lack of improvement (Voulvoulis et al. 2017).
A friendly explanation for the lack of progress is that improvements are sometimes masked by the way the status is assessed, because a poor assessment of one ecosystem component results in an overall poor assessment of the river reach (one out rule). Also, slight improvements may have occurred that are insufficient for reaching a higher status class.
Possibly, the time since the implementation of restoration and mitigation measures has been too short to achieve a full recovery of the ecological system. However, research suggests that time may not be the main explanation for the absence of recovery in many cases. For instance, not the time since the implementation of restoration measures but the distance to recolonization pools in healthy ecosystem were the main predictor of the success of restoration measures (Wilking et al. 2016, Tonkin et al. 2014, Leps et al., 2016). Unfortunately, pristine ecosystems have become rare, as indicated by the widespread poor status of ecological communities, complicating the improvement of the ecological status in many regions.
Moreover, as stated above, the poor ecological status is the result of multiple interacting stressors, where pollution with nutrients, pesticides, and other chemicals could still be more important than currently acknowledged. Many of these stressors are related to agriculture and the implementation of riparian buffer strips adjacent to agricultural fields can represent an effective mitigation measure. These buffer strips reduce pesticide (Reichenberger et al 2007, Schulz 2004), as well as nutrient and sediment input, and simultaneously provide shading as a beneficial side effect in the light of global warming (Feld et al. 2018). However, their specific design in terms of width, length, position in the catchment, and vegetation depends on the stressor the mitigation measure targets.
Another option to reduce the pollution of surface water is the upgrading of wastewater treatment plants, which are considered to be the main input path for micropollutants, such as pharmaceuticals, into streams. Such an upgrade could be achieved through the implementation of an additional (typically fourth) cleaning step that relies on activated charcoal or ozonisation. Several pilot studies in Switzerland and Germany (VSA 2018) demonstrated a general improvement of water quality. For example, a study in a real wastewater treatment plant showed that additional treatment step strongly increased the removal rates of micropollutants (Margot et al. 2013). Likewise ecotoxicological effects on algae and fish were significantly reduced through additional treatment steps. These results have been confirmed in other studies (Fux et al. 2015, Bourgin et al. 2018). In Switzerland, it is therefore mandatory since 2016 to eliminate organic micropollutants in large waste water treatment plants as well as in wastewater treatment plants that discharge into the catchment areas of lakes or that account for a high percentage of the receiving catchment’s discharge. However, additional treatment comes at additional costs and energy consumption: For Switzerland an increase in costs of 10-25 percent and an increase in energy consumption of 10-20 percent was estimated (Abegglen 2011). Nevertheless, such end-of-pipe technology is often easier to implement compared to more complex solutions for reducing various point and non-point sources of micropollutants, such as reducing the unwanted side-effects of intensive agriculture while maintaining yields or preventing the disposal of chemicals by individuals into the sewage system. Nevertheless, end-of pipe solutions treat symptoms rather than causes and may be insufficient to completely solve environmental problems in the long run.
The beauty of the WFD is that its original focus was on an in-depth understanding of interdependent human-environment interactions, and a river basin management that involves stakeholders and considers conflicting water uses as opposed to a focus on uni-dimensional end-of pipe solutions. However, Voulvoulis et al. 2017 argue that the implementation of the WFD has departed from this focus and abandoned the great aspirations, which may be the main reason for its failure to deliver on the widespread improvement of the status of surface water bodies. Consequently, the most important road to success may be a re-orientation in terms of a fundamental shift towards systems thinking and adoption of interdisciplinary, integrated and holistic approaches for water managment, where changes in agricultural policies are as important as localized river restoration and where, most importantly, the selected restoration and mitigation measures are adapted to the individual catchment and its specific socio-economic context.
Ralf B. Schäfer is professor for Quantitative Landscape Ecology and studies the responses of freshwater microorganisms and invertebrates as well as of freshwater ecosystem functions to stressors such as chemicals, climate change and land use. He has lead several national and international projects and published several influential papers on these topics.
Mira Kattwinkel is a scientist at the University of Koblenz-Landau in the group Quantitative Landscape Ecology. She works on the effects of anthropogenic stressors, mainly pesticides, on aquatic communities using statistical as well as dynamic models. Additionally, she develops tools for GIS data processing and Bayesian parameter inference.
Elisabeth Berger is a researcher at the University of Koblenz-Landau in the group Quantitative Landscape Ecology. She studied marine biology and environmental sciences and received a PhD in freshwater ecology from the University of Frankfurt, where she focussed on stressor prioritisation for water management.
References:
Abegglen, C., Beier, S., Pinnekamp, J., Mauer, C., Siegrist, H. 2011 Mikroverunreinigungen: Energieverbrauch und Kosten weitergehender Verfahren auf kommunalen ARA. Gas, Wasser, Abwasser 7, 479-486.
Adler R.W. 2017 The decline and (possible) renewal of aspiration in the Clean Water Act. Washington Law Review 88, 55.
Borchardt D., Mohaupt V., Jekel M. & Rohrmoser W. 2013 Die Wasserrahmenrichtlinie Eine Zwischenbilanz zur Umsetzung der Maßnahmenprogramme 2012. Bundesministerium für Umwelt, Naturschutz und Reaktorsicherheit (BMU), Berlin.
Bourgin, M., Beck, B., Boehler, M., Borowska, E., Fleiner, J., Salhi, E., Teichler, R., von Gunten, U., Siegrist, H., and McArdell, C.S. 2018 Evaluation of a full-scale wastewater, treatment plant upgraded with ozonation and biological post-treatments: Abatement of micropollutants, formation of transformation products and oxidation by-products. Water Research 129, 486–498.
EC (European Commission) 2000 Directive 2000/60/EC of the European Parliament and of the Council of 23 October 2000 establishing a framework for Community action in the field of water policy.
EEA (European Environment Agency) 2018 European waters Assessment of status and pressures 2018. Publications Office of the European Union, Luxembourg. https://www.eea.europa.eu/publications/state-of-water/at_download/file
Fantke P., Weber R. & Scheringer M. 2015 From incremental to fundamental substitution in chemical alternatives assessment. Sustainable Chemistry and Pharmacy 1, 1–8.
Feld C.K., Fernandes M.R., Ferreira M.T., Hering D., Ormerod S.J., Venohr M., Gutierrez-Canovas C. 2018 Evaluating riparian solutions to multiple stressor problems in river ecosystems – A conceptual study. Water Research 139, 381–394.
Fux C., Kienle C., Joss A., Wittmer A., Frei R. 2015 Ausbau der ARA Basel mit 4. Reinigungsstufe. Pilotstudie: Elimination Mikroverunreinigungen und ökotoxikologische Wirkungen. Aqua & Gas 95, 10–17.
Hering D., Feld C.K., Moog O. & Ofenbock T. 2006 Cook book for the development of a Multimetric Index for biological condition of aquatic ecosystems: Experiences from the European AQEM and STAR projects and related initiatives. Hydrobiologia 566, 311–324.
Leps, M., Sundermann, A., Tonkin, J.D., Lorenz, A.W., Haase, P., 2016. Time is no healer: increasing restoration age does not lead to improved benthic invertebrate communities in restored river reaches. Science of The Total Environment 557–558, 722–732.
Malaj E., von der Ohe P.C., Grote M., Kühne R., Mondy C.P., Usseglio-Polatera P., Brack W. & Schäfer R.B. 2014 Organic chemicals jeopardize the health of freshwater ecosystems on the continental scale. Proceedings of the National Academy of Sciences 111, 9549–9554.
Margot J., Kienle C., Magnet A., Weil M., Rossi L., de Alencastro LF., Abegglen C., Thonney D., Chèvre N., Schärer M., Barry D.A. 2013 Treatment of micropollutants in municipal wastewater: Ozone or powdered activated carbon? Science of The Total Environment. 461–462, 480–498.
Mondy C.P., Villeneuve B., Archaimbault V. & Usseglio-Polatera P. 2012 A new macroinvertebrate-based multimetric index (I2M2) to evaluate ecological quality of French wadeable streams fulfilling the WFD demands: A taxonomical and trait approach. Ecological Indicators 18, 452–467.
Palmer, M.A., Menninger, H.L., Bernhardt, E., 2010. River restoration, habitat heterogeneity and biodiversity: a failure of theory or practice? Freshw. Biol. 55, 205–222.
Reichenberger, S., Bach, M., Skitschak, A. & Frede, H. G. 2007 Mitigation strategies to reduce pesticide inputs into ground- and surface water and their effectiveness; A review. Science of the Total Environment 384, 1–35.
Schäfer R.B., Kühn B., Malaj E., König A. & Gergs R. 2016 Contribution of organic toxicants to multiple stress in river ecosystems. Freshwater Biology 61, 2116–2128.
Schmidt-Kloiber A., Moe S.J., Dudley B., Strackbein J. & Vogl R. (2012) The WISER metadatabase: the key to more than 100 ecological datasets from European rivers, lakes and coastal waters. Hydrobiologia 704, 29–38.
Schinegger R., Palt M., Segurado P. & Schmutz S. 2016 Untangling the effects of multiple human stressors and their impacts on fish assemblages in European running waters. Science of The Total Environment 573, 1079–1088.
Schulz, R. 2004 Field studies on exposure, effects, and risk mitigation of aquatic nonpoint-source insecticide pollution: A review. Journal of Environmental Quality 33, 419–448.
Tonkin J.D., Stoll S., Sundermann A., Haase P. 2014 Dispersal Distance and the Pool of Taxa, but Not Barriers, Determine the Colonisation of Restored River Reaches by Benthic Invertebrates. Freshwater Biology 59, 1843–55.
US (United States) Congress 1972 Federal Water Pollution Control Amendments of 1972.
VSA, Verband Schweizer Abwasser- und Gewässerfachleute 2018 https://www.micropoll.ch/de/anlagen-projekte (accessed 19.10.2018)
Vorkamp K. & Sanderson H. 2016 European environmental quality standards (EQS) variabiliy study: Analysis of the variability between national EQS values across Europe for selected water framework directive river basin-specific pollutants. DCE – Nationalt Center for Miljø og Energi, Aarhus University, Aarhus. http://dce2.au.dk/pub/SR198.pdf
Voulvoulis N., Arpon K.D. & Giakoumis T. 2017 The EU Water Framework Directive: From great expectations to problems with implementation. Science of The Total Environment 575, 358–366.
Winking C., Lorenz A.W.,, Sures B., Hering D. 2016 Start at Zero: Succession of Benthic Invertebrate Assemblages in Restored Former Sewage Channels. Aquatic Sciences 78, 683–94.
Photo: Aquarius | Shutterstock
Published on December 5, 2018.